You are here: Agriculture > Transgene Plants > Plant protection > Releases
4 Releases and Commercialization
4.1 Safety Aspects
In the early 1970s, the introduction of molecular gene transfer technologies
in bacteria gave rise to questions among scientists about possible risks
of this new technology. Following the first successful gene transfer in
1975, the scientific community discussed these questions on the conference
of Asilomar and decided to set up rules for the self-control of laboratory
experiments in order to avoid any inadvertent generation of harmful effects.
This resulted in a number of national and international recommendations,
guidelines and legislations, e.g. the OECD report Recombinant DNA Safety
Considerations (1986). With the accumulation of experience with transgenic
organisms and the better understanding of genetics and gene transfer,
among scientists the opinion began to prevail that genetic engineering
itself does not pose specific novel risks and that regulatory review should
focus on the hazard potential related to characteristics of the transformed
organism and the introduced traits, not on the process by which it has
been created. No accidents or other deleterious effects of transgenic
plants or other transgenic organisms have been reported so far. However,
in the public there is still concern about the risks of genetic engineering,
sometimes leading to complete refusal of any application of this technique.
In the following, general safety aspects related to the release of
transgenic crops and specific safety aspects of the methods used to achieve
disease or pest resistance are reviewed.
4.1.1 Gene Transfer
4.1.1.1 Outcrossing to other Plants
Genes may be transferred by pollen to other cultivars of the same crop
or, more rarely, to wild or weedy relatives that grow in the agricultural
or natural environment. The chances for movement of genes from a crop to
a wild relative depend on a number of coincidences. These events are as
follows.
- A sexually compatible plant grows within the range of movement of the
transgenic pollen;
- the related plant forms receptive flowers at or near the time of movement
of transgenic pollen;
- a flower of the related plant is fertilized and viable seed is produced;
- the seeds germinate and grow and
- the resulting plant and its progeny are fertile, i.e. can produce seed
by self- or cross-pollination, or can survive vegetatively.
[38]
If one of these events does not occur, outcrossing is not an event of
concern. Gene transfer by pollen to a wild relative within a population
can be considered as analogous to a mutation arising in the population.
Whether the transferred trait becomes initially established in the
population depends more on chance effects than on fitness, since the
great majority of mutants are lost from the population because of genetic
drift, even if the gene confers specific advantages (Gale 1990, cited in
[38]. Only repeated pollination will lead to the integration
of the trait into the wild genetic background. Whether the new trait will
spread in the population or get lost again depends on the effect the
transferred gene has on the wild relative. If the effect confers a
selective advantage on the hybrid plant under the prevailing environmental
conditions, the hybrid plants may spread and could cause changes in the
species composition. Selective advantages might be caused by greater
resistance to a disease or an insect pest, or greater tolerance to
environmental stresses present in the agricultural or natural environment.
[38]
A weed may be defined as "a plant which is not intentionally sown, whose
undesirable qualities outweigh its good points" (Granatstein 1989, cited
in [39], although several other definitions exist. A wild
relative that becomes a weed because of the introduced trait, or a weed
with an increased weediness due to the introduction of a transgene by
outcrossing with a transgenic crop may be identified as a safety concern.
Therefore, measures must be taken to prevent outcrossing of genes which
could increase the fitness of wild relatives, if it is possible that all
the above mentioned conditions are met simultaneously. These measures may
include isolation of test sites from areas inhabited by relatives,
control of pollen sources by mowing or herbicides, using sterile plant
lines unable to produce seeds in the case of clonally propagated plant
lines, and others. [38] The recently developed method of plastid
transformation (see chapter 3.1.1) offers advantages for the containment
of transgenes. In plastid transformation, the transgene is introduced
into the genome of the plant chloroplasts. As chloroplasts are inherited
maternally only in almost all crop species, pollen produced by plants
carrying the transgene in the plastid genome do not contain the transgene.
[11]
4.1.1.2 Gene Transfer to Microorganisms
As discussed above, Agrobacterium tumefaciens is a widely used bacterial
vector in plant genetic engineering. Some Agrobacteria carrying the
transgene may be present in plant cells after transformation and could
exit through the roots into the soil, where the transgene could be passed
on via conjugation to other bacteria or to other plants via infection.
Therefore, the absence of Agrobacteria is an important requirement for
the release of transgenic plants into the environment.
Another possible mechanism for gene transfer is the uptake of DNA released
by decaying plants through microorganisms. However, for this "horizontal
gene transfer" event to occur, several obstacles would first have to be
overcome.
The DNA has to remain at least partly intact in the soil. It was shown
that the absorption of DNA on sand particles made the DNA less vulnerable
to degrading DNases.
Foreign DNA taken up by bacteria is usually rapidly degraded by restriction
enzymes. This mechanism protecting microorganisms against viruses can be
interrupted only if the invading DNA is modified very quickly so that its
methylation pattern resembles that of the bacteria.
DNA can only be established in the bacterial cell and inherited if it is
coupled to an bacterial origin of replication. Therefore, the DNA has to
be incorporated into the bacterial genome by homologous recombination,
or - if the transgene already contains a bacterial origin of replication -
must become ligated to a circular plasmid. [40]
Successful transfer of a functional gene must be considered a very rare
event. As in the case of outcrossing to related plants, the transferred
gene will only be maintained in the bacterial population if it confers a
selective advantage, which makes successful horizontal gene transfer even
more unlikely. However, evolutionary considerations indicate that gene
transfer from plants to bacteria may have taken place, as some bacterial
enzymes are known to have a sequence more similar to eucaryote sequences
than to procaryotic types. Another possible type of horizontal gene
transfer, viral recombination with transgenic plant mRNA transcripts,
is discussed in chapter 4.1.4. [40] BATS-Report 3/94
(Tools for Safety Assessment. The release of transgenic plants Horizontal gene transfer)
[41] reviews the latest literature concerning horizontal gene
transfer to microorganisms.
4.1.2 Increased Weediness
A crop is considered a weed when it carries over (grows in subsequent
seasons) or establishes in neighboring fields and competes with subsequent
crops. Most crop species depend completely on human nurturing and are
therefore unable to compete successfully with plants adapted to the
natural environment. There are, however, examples of crops that are minor
weeds in natural or agricultural ecosystems, examples being oilseed rape,
sunflower and rye. According to Baker (1974, cited in [38]), weediness
is a multicharacter attribute and the addition of one gene is unlikely
to cause a crop to become a weed. In contrast, Fitter et al. (1990) and
Williamson et al. (1990, cited in [38]) suggest that the
alteration of one gene may indeed be enough to change a crop into a weed.
If a crop species has very few weedy characteristics, the addition of
one or a few genes would be unlikely to cause that crop to become a weed
problem. Special attention might be warranted where the crop has weedy
characteristics or the added genes might be expected to improve the crops
competitive ability in natural or agricultural ecosystems.
[38]
An indication of the tendency of certain cultivars to carry over may be
obtained from previous experience (familiarity) with the crop, the
introduced trait, the environment in which the plant will be grown, and
their interactions. Standard agricultural practices like tillage, mowing,
herbicide use and crop rotation are widely available for the control of
weeds within the agricultural environment, including the control of
carry-over of transgenic plant lines at the test site.[38]
4.1.3 Undesirable Phenotypic Traits
Presently, introduced genes can only be targeted to a predicted site by
using the recently developed method of plastid transformation. Therefore,
in most experiments the transgene integrates randomly into the plant
genome. The introduction of foreign genes effects the plant metabolism
directly through the new gene product, as well as through possible
secondary interactions. Direct effects of the transgene products will be
described in the chapters dealing with the different methods designed to
achieve pest resistance. Here, only possible secondary effects of the
transgene integration are discussed. Such secondary effects may arise
from the interruption of plant genes resulting from the integration of
the transgene, or changes in the expression rate of other genes caused by
the introduction of new promoters and position effects. However, in this
respect, transgenic plants are not basically different from products of
traditional plant breeding, where secondary effects are known as well.
One example is brown sorghum bred for bird-resistance, where a higher
tannin content caused anti-nutritional properties of the seeds. Another
example is a potato variety bred for resistance to the Colorado potato
beetle which contained to much solanin, and was therefore not registered
by the German Federal Cultivar Office. [40]
As no predictions of secondary effects of new plant varieties (whether
obtained by traditional breeding or with the help of genetic engineering)
are possible, investigations have to be done after hybridization or gene
transfer. Morphology, growth, yield, nutritional composition, and levels
of potential toxins are assessed during development of a new variety,
where only plants without undesirable phenotipical traits will be selected
for further breeding. [40]
4.1.4 Specific Safety Aspects
4.1.4.1 Strategies against Insects
Bacillus Thuringiensis Toxin
Bacillus thuringiensis (Bt) has been used as a biological pesticide for
more than 50 years now, apparently without any deleterious effects on non-
target insects, other animals and humans. [8] The spectrum of
activity of an individual δ-endotoxin tends to be quite narrow,
with a given δ-endotoxin being active only against a few (known)
insects. Solubilization in the insect midgut, activation of the protoxin
and toxin binding to the receptor in the midgut are steps which play a
role in the specificity of a δ-endotoxin. [8] Possible
toxic effects of Bt toxin expressed in transgenic plants have also been
intensively investigated. No effects on humans or other mammals were found.
For instance, rats were fed with the Bt toxin CrylA(b) corresponding to
a human daily consumption of 2000 kg transgenic Bt-expressing tomatoes.
No damage could be observed. Also a 90-day feeding trial with rats did
not reveal any signs of adverse effects. [42]
Several major pest species have shown their ability to adapt to Bt toxins,
either in laboratory tests or in the field. Changes in the specificity of
the toxin receptor seem to represent the major cause of resistance
development. Insects are well known for their ability to develop resistance
against insecticides and the development of resistance to Bt toxins is not
specific for the expression of Bt toxin in transgenic plants. Different
strategies are discussed for the management of insect resistance, when
deploying Bt toxins through transgenic plants, e.g. developing and
maintaining refuges for the survival of susceptible insects, growing
mixtures of cultivars expressing different toxins, sequentially planting
such different cultivars, expressing mixtures of toxins in one transgenic
plant line, etc.. None of these strategies have proven their general
suitability, and extensive field trials are necessary to further evaluate
their advantages and disadvantages. [43]
Protease Inhibitors, Amylase Inhibitors, and Lectins
Protease and amylase inhibitors apparently act by interfering with the
insect digestive process. The conditions under which digestive processes
take place differ considerably between insects and mammals and between
different orders of insects, e.g. regarding midgut pH. Therefore, protease
inhibitors affecting insect enzymes would not necessarily also effect
enzymes of human consumers. Cowpea trypsin inhibitor (CpTI) e.g. apparently
lacks mammalian toxicity.[15] However, these inhibitors may
also become harmful to beneficial insects if they are constitutively
expressed at the levels necessary to accomplish protection, and their
potential impact must therefore be assessed carefully before large-scale
field trials take place. [9]
Protease inhibitors, amylase inhibitors and lectins are examples of proteins
which are part of the natural plant defense system. They are therefore
already present in our daily diet. However, most (genetically unaltered)
plants contain substances which can be toxic to humans or other consumers
or which cause allergies to susceptible people. For instance, lectins are
known to be toxic to both mammals and birds when ingested without heating
prior to consumption.[16] Therefore, it could still be possible
that such insecticidal substances cause harm to consumers, especially if
they are produced in higher amounts than usual in transgenic plants. As
the products of the transgene are known, the toxic and allergenic effects
can be evaluated prior to a potential commercialization. This makes sure
that transgenic crops reaching the marketplace are as safe as other food
sources.
4.1.4.2 Strategies against Viruses
Viral Coat Proteins
In coat protein-mediated protection (CPMP), resistance to viruses is
achieved by expression of a viral coat protein in transgenic plants. A
potential adverse effect of CPMP comes from the possibility of
heterologous encapsidation (also called transcapsidation or
heteroencapsidation) resulting from the following mechanism: When a
transgenic plant synthesizing a viral coat protein is infected with
another virus, the replicated nucleic acid of the second virus may be
packed (encapsidated) into the viral coat produced by the plant. Because
encapsidation is a relatively specific phenomenon, heterologous
encapsidation is unlikely except for closely related viruses or strains
of viruses. As the coat protein plays an essential role in virus
transmission by vectors such as aphids or nematodes, the proposed
mechanism could affect the transmission properties of the infecting virus
Indeed, this effect could be shown in the laboratory with transgenic
tobacco plants expressing the coat protein of an aphid transmissible
plum pox potyvirus (PPV) strain. After infection with an aphid-
nontransmissible zucchini yellow mosaic virus (ZYMV) strain, aphid-
transmissible PPV coat containing ZYMV genome was found, and the
combination was found to be aphid-transmissible. [23]
A situation possibly leading to heteroencapsidation also exists in nature,
when a plant cell is infected by two viruses simultaneously. In this
case, the genome of one virus could be packed into the coat protein of
the second. However, it is important to note that heterologous
encapsidation does not lead to a change in the viral genome and therefore
is a "one generation problem". New viral transmission properties - if any
- would be lost again after one infection cycle and not inherited to
subsequent generations. [23]
Another potential environmental risk could arise from the recombination of
viral RNA with plant messenger RNA (mRNA) from genetically engineered
viral coat protein genes. Viral recombination could lead to viruses with
new traits, e.g. altered virulence. A mechanism for viral recombination
has been termed copy choice or template switching and is thought to be a
quite general phenomenon, although not involved in the normal replication
circle. In nature, there is evidence for incorporation of sequences of
plant or animal RNA in plant and animal viruses. For example, the genome
of the S-strain of potato leaf roll virus contains a 119-nucleotide
segment of a plant chloroplast mRNA. There is at this time only one case
described of plant viruses in which natural incorporation of host
sequences may have led to changes in virus pathogenicity. [23]
Viral recombination in transgenic plants could be shown in a recent
laboratory experiment. [44] Whether large-scale cultivation
of transgenic plants could increase the frequency of viral recombination
or heterologous encapsidation is not known. [23]
Other Virus-Derived Genes
As the other approaches to achieve virus resistance do not lead to
production of a coat protein, no heteroencapsidation is possible. Several
examples have been described where high levels of resistance with low
expression rates of defective viral RNAs or antisense RNA without
production of functional proteins has resulted in high levels of
resistance. These strategies are unlikely to cause risks higher than in
non-transgenic plants.
Satellite RNA
Certain satellite RNAs of cauliflower mosaic virus induce a lethal necrosis
in tomato and certain related species. Outbreaks of lethal necrosis are
usually limited, but have reached epidemic proportions in Italy in 1988
and 1989. Sequence differences between satellite RNAs that reduce disease
symptoms and those that cause lethal necrosis can be very small. In some
cases, a single point mutation caused a nonnecrogenic satellite RNA to
become necrogenic in tomato. [23] Therefore, the satellite RNA strategy
is thought to be associated with risks and needs further investigation.
[9]
4.1.4.3 Strategies against Fungi and Bacteria
The genes used for resistance against fungi mostly come from plants and
their products are therefore not new in our diet. This does not
necessarily mean that they cannot cause problems, as already discussed
in chapter 4.1.3. Careful assessment of toxicity and allergenic potential
prior to large-scale cultivation and use as human or animal food can
ensure that transgenic crops are as safe as conventional crops. Basically
the same is true for genes which are derived from organisms not used as
food, e.g. cecropin genes from insects against bacteria. However, the
products of genes which are not normally present in food may need an even
more careful evaluation, because less experience with these compounds
exists.
4.1.4.4 Transgenic Baculoviruses
Baculoviruses have been genetically altered to decrease the time they need
to kill plant-feeding insects. Effects on non-target organisms like other
insects or humans and spread of transgenic baculoviruses have been in the
center of interest. Baculoviruses were found to be non-pathogenic to
mammals, other vertebrates, and plants, and are unable to penetrate
through nuclei of mammalian cells. Most baculoviruses are active only on
a single insect family or genus. The restricted host range strongly reduces
the risks of deleterious effects on beneficial and non-target organisms.
For field releases, transgenic viruses with a selective disadvantage
relative to the wild-type can be used, so that recombinant strains are
rapidly competed out by wild-type viruses, if application of transgenic
viruses is discontinued. The shorter time to kill of transgenic viruses
is also a selective disadvantage, because less new viral particles are
produced before the host insect dies. [33] However, repeated
commercial applications of transgenic baculoviruses at a single site may
lead to high levels of viruses in the soil, and the ecological
consequences of this are currently unclear. [45]
4.1.4.5 Transgenic Bacteria
Inactivated Bacteria Expressing Bt Toxin
The genes for the δ-endotoxin of Bacillus thuringiensis (Bt toxin)
have been transformed into Pseudomonas fluorescens to avoid the rapid
degradation of Bt toxin which occurs when Bacillus thuringiensis is used
as an insecticidal spray. Since the transgenic P. fluorescens are killed
before spraying and no living transgenic microorganisms are released into
the environment, this approach has the advantage of relative freedom from
environmental and safety concerns associated with field releases of living
transgenic organisms. In 1985, the U. S. Environmental Protection Agency
(EPA) approved testing of the inactivated P. fluorescens with the first
field release permit for a transgenic product. [36]
Bacterial Endophytes Expressing Bt Toxin
In order to increase the effect of Bt toxins, the corresponding genes have
also been introduced into bacterial endophytes (bacteria that live inside
of plants). As these endophytes produce the Bt toxin inside the plant,
the toxin is also effective against insect pests feeding inside the plant,
and it is not quickly degraded like Bt toxin sprayed on plants.
Clavibacter xyli spp. cynodontis (Cxc) has been used for Bt toxin delivery
in the most advanced approach. Most of the available information about
the biology of Cxc has been generated since 1986 in extensive laboratory,
greenhouse, and field studies performed by the company Crop Genetics
International (CGI) and cooperators for submission to the U.S.
Environmental Protection Agency (EPA) and the Animal and Plant Health
Inspection Service (APHIS) in support of development and registration of
products for maize and rice protection. [37]
These studies show that in nature a living host is required for replication
of Cxc, that Cxc is short lived in maize plant debris and cannot be
detected in soil or irrigation runoff water around inoculated plants.
Volunteer maize seedlings originating from inoculated plants did not
become colonized. Xylem-feeding leafhoppers and flea beetles, both of
which are known vectors of bacterial pathogens of the plant vascular
system, and several other tested insect species, were found to be unable
to transmit Cxc to uninfested plants. The results of these studies have
provided evidence that soil, water, colonized plants, plant debris and
insects are unlikely to serve as a source of persistent Cxc inoculum for
weeds, neighboring fields or subsequent crops in the same field.
Apparently, Cxc is transmitted in nature by vegetative propagation of
bermudagrass via runners, and only through mechanical plant injury
(animal grazing, mowing) does it occasionally move to other plants.
[37]
The engineered genes are spontaneously lost from Cxc at low frequency due
to homologous recombination with sequences originally present on the Cxc
chromosome. The resulting segregants (cells which have lost the introduced
trait) appear to be identical to the wild-type Cxc strain. Segregants
grow more quickly than recombinants, so that selection eventually leads
to complete loss of bacteria containing the recombinant genes. The progress
of this reversion phenomenon is sufficiently low to ensure product
performance within a growing season, but occurs rapidly enough to insure
that Bt toxin genes would not persist in the Cxc population.
[37]
4.2 Regulatory Framework
4.2.1 Case Study USA
U.S. federal policy does not view genetically engineered organisms as
fundamentally different from those traditionally isolated from nature and
introduced into new environments, or varieties produced through programs
of breeding and selection. Not the technique by which certain traits have
been engineered into an organism is in the focus of the regulation, but
the exclusion of hazards resulting from the engineered organism.
Consequently, the release and commercialization of transgenic organisms
is regulated under existing laws, which have been partially adapted to
the new techniques. The three main administrations concerned with
transgenic organisms are the U.S. Department of Agriculture (USDA),
the Environmental Protection Agency (EPA) and the Food and Drug
Administration (FDA). In short, the USDA deals with plant pest traits
which may be exhibited by the genetically altered organism, the EPA
investigates primary and secondary effects of potentially toxic compounds
(especially pesticides) expressed by transgenic organisms, and finally,
the FDA analyses other compounds not covered by the EPA. The three
departments work together in order to minimize duplications of efforts
in areas of overlap such as the assessment of environmental data and the
preparation of environmental documentation. In the following, the different
focuses and aims of these organizations are described in more detail.
4.2.1.1 U.S. Department of Agriculture
The U.S. Department of Agriculture (USDA) has a statutory mandate to
protect U.S. agriculture against the introduction and dissemination of
plant pests. Under the Federal Plant Pest Act (FPPA) and the Plant
Quarantine Act (PQA), the Animal and Plant Health Inspection Service
(APHIS), which is the regulatory arm of the USDA, regulates the movement
into and through the United States of plants, plant products, plant pests,
and any product or article that may contain a plant pest at the time of
movement.
In the FPPA, a plant pest is defined as "any living stage of any insects,
mites, nematodes, slugs, snails, protozoa, or other invertebrate animals,
bacteria, fungi, or parasitic plants or reproductive parts thereof,
viruses, or any organisms similar to or allied with any of the foregoing,
or any infectious substances, which can directly or indirectly injure or
cause disease or damage in any plants or parts thereof, or any processed,
manufactured, or other products of plants".
Genetically engineered organisms are deemed "regulated articles" under the
regulations if the gene donor organism, the recipient organism, or the
vector organism meets the definition of plant pest. The focus of the
review under the regulations is to certify that the there is no plant
pest risk from shipping or field testing the organisms even though the
organism was developed through the use of genetic material from a plant
pest. As many plants are transformed using the "disarmed" plant pest
Agrobacterium as a vector, and/or use genes derived from pathogens (like
viral coat proteins), virtually all transgenic plants fall under the
APHIS regulations.
To apply for a field test permit for a genetically engineered organism
under the regulations, an applicant must complete an APHIS form and supply
the information requested in the form. A permit application must contain
sufficient information on the crop plant, the nature of the genetic
modification, and the protocol for conducting the field trial to allow
for an evaluation of any potential plant pest risk or environmental
effects that may result from the field trial. To assist applicants in
preparing an application, a user's guide has been developed that provides
a detailed description of the elements to consider in writing an
application. [46] The application should be submitted at least
120 days in advance of the proposed field test. Within 30 days after the
application is received by APHIS, a copy of a preliminary assessment is
sent to the state in which the test will take place, and the state
agencies have 30 days after receiving the application to comment.
A key component of the permit review process is the environmental assessment
(EA), which contains a thorough accounting of the agency's analysis
leading to a decision on whether to issue a permit. If the environmental
assessment results in a finding of no plant pest risk and of no significant
impact on the environment, the permit is issued. The EA is a public document
that provides assurance that APHIS has fully considered the possible
consequences of releasing the regulated article into the environment. EAs
are available via Internet (see [16] for further information).
Unlike the EPA, APHIS has no licensing authority to regulate the commercial
use of genetically engineered products, but the "delisting" of the product
as a plant pest is an important prerequisite for commercialization.
[47]
4.2.1.2 Environmental Protection Agency
Three of the statutes administered by the U. S. Environmental Protection
Agency (EPA) address the environmental and human health issues associated
with the use of pesticides and other products that enhance agricultural
production: The Federal Insecticide, Fungicide, and Rodenticide Act
(FIFRA), the Federal Food, Drug and Cosmetic Act (FFDCA), and the Toxic
Substances Control Act (TSCA). Under FIFRA, the EPA has the authority to
regulate development, sale, use, and disposal of pesticides. Pest control
substances produced by genetically engineered plants or microorganisms
are pesticides within the meaning of FIFRA and are therefore subject to
FIFRA. To date, most transgenic organisms regulated under FIFRA express
Bacillus thuringiensis δ-endotoxins. For a pesticide to be registered,
FIFRA requires that the pesticide will not, when used in accordance with
commonly recognized practice, cause "unreasonable adverse effects", taking
into account 1he economic, social, and environmental costs and benefits
of the use of [the] pesticide".
Under the Federal Food, Drug, and Cosmetics Act (FFDCA), EPA is responsible
for determining the amount of pesticide that may be present in raw or
processed agricultural commodities when they enter commerce. The statute
gives broad authority to protect against human dietary risks that might
be posed by the use of pesticides (including pesticides produced by
transgenic organisms) in food for humans or as feed for animals. FFDCA
contains a concept that some substances in food may be "generally recognized
as safe" (GRAS). A tolerance (the maximum level of pesticide residue
allowable in a food or feed) is not required if a substance/pesticide is
GRAS. GRAS status is based either on a safe record of use in food or
evidence of safety and widespread agreement in the appropriate scientific
community.
The Toxic Substances Control Act (TSCA) authorizes EPA to acquire
information on chemical substances and mixtures of chemical substances in
order to identify and regulate potential hazards and exposures. EPA is
required to screen new chemical substances prior to their introduction
into commerce to identify unreasonable risks to human health or the
environment. If EPA takes no action, the submitter may proceed to
commercialization, and the substance may be listed in the inventory.
Substances listed in the inventory are not new. Organisms, especially
microorganisms, are viewed as mixtures of chemical substances in the TSCA
and may therefore be subject to TSCA. EPA interprets a "new, microorganism
to be one formed by deliberate combination of genetic material from source
organisms classified in different taxonomic genera, also called "intergeneric
microorganisms". An example of transgenic organisms that come under the
authority of TSCA are nitrogen-fixing bacteria of the genus Rhizobium.
[48]
4.2.1.3 Food and Drug Administration
The Food and Drug Administration (FDA) issued a policy statement on foods
derived from new plant varieties, including genetically engineered plants,
in 1992. This document stated that most substances added to food as a
result of genetic modification are substantially similar to substances
commonly found in food and therefore should not be subject to premarket
"food additive" regulation under FDCA unless "objective characteristics
raise questions of safety sufficient to warrant formal premarket review
and approval." The implication of the document is that most substances
added to food as a result of genetic modification would be considered as
"generally recognized as safe" (GRAS) and would not require regulatory
approval as a food additive. The FDA food policy establishes that
substances in plants that properly meet the definition of pesticide under
FIFRA will be addressed by the EPA. [47]
4.2.2 Case Study European Union/Germany
The situation in the European Union (EU) is more complex than in the USA,
as the EU consists of a number of independent states which have agreed to
form a single market. They have conceded some of their powers to the EU,
but essentially still operate as independent Member States. The Council
of Ministers, which is the final decision-making body of the EU, has
issued several directives concerning genetic engineering. Directives are
binding as to the results to be achieved, but the form and the methods
used to achieve the results are left to the national authorities who
translate them into national law. [49]
4.2.2.1 European Union Directive 901220/EC
The main directive concerning the release of genetically engineered plants
is the "Council Directive on the Deliberate Release into the Environment
of Genetically Modified Organisms", known as "90/220/EC". The directive
was adopted in 1990 and covers all genetically engineered microorganisms,
animals and plants through all stages of release. All deliberate releases
must be reviewed on a step-by-step, case-by-case basis and must be
accompanied by an environmental impact assessment. Releases can only take
place where a national approval procedure for experimental release already
exists.
There are considerable differences in how the member states have incorporated
directive 90/220/EC into national law, and how this laws are interpreted.
In many countries, the regulatory activity has been based on the premise
that genetic engineering is a technique in its own and that there are
unique products and of biotechnology which require special considerations.
Consequently, some countries, e.g. Germany, have established a special
genetic engineering act. This is in contrast to the situation in the USA
described above, where not the fact that an organism has been genetically
engineered, but the (novel) traits of the modified organism are in the
focus of regulation. [49] As an example of a country with a genetic
engineering act, the situation in Germany will be described in more
detail.
4.2.2.2 Releases under the German Genetic Engineering Law
The German genetic engineering law (GenTG, Gesetz- zur Regelung von Fragen
der Gentechnik) forms a binding framework for genetic engineering activities
in Germany. Paragraphs 14-16 and 18 describe the procedures which must be
followed for deliberate releases of transgenic organisms. The ZKBS (Zentrale
Kommission for biologische Sicherheit, central biosafety committee)
scrutinizes and evaluates the applications for releases. The ZKBS asks
for the comments of the BBA (Biologische Bundesanstalt, federal biological
institute), the Umweltbundesamt (federal environmental agency) and the
agencies of the Bundeslaender (federal states) in which the field release
will take place. If all legal conditions are met (e.g. all information
required submitted), the application should be answered within three
month . [49]
The documents required for the application include the following data:
[50]
Information on gene donor, gene recipient, vector and transgenic
organism. These cover taxonomic data, phenotypic and genotypic traits,
and methods for identification. All possible risk factors like pathogenic
traits, toxins or allergens produced, possible gene transfer mechanisms,
potential for survival or spread, and so on, must be named here. The
information about the transgenic organism must contain additional data
on the number of transgenes introduced, transgene expression and stability,
detailed description of the transgene, its products, and experiences with
the transgenic organism in laboratory or greenhouse experiments.
Description of the site and its surroundings, like location of the site,
beginning and end of release, area, number of transgenic organisms released,
inactivation of transgenic organisms at the end of the trial, workers safety,
information on the environment (other plants, animals, endangered species
nearby, villages), climate, soil, and so on.
The most difficult part of the application deals with possible interactions
between the transgenic organism and the environment. The details required
include information on potential survival and spread, possibility of gene
transfer, selective advantages, effects on non-target organisms, etc..
The last part of the application deals with monitoring, waste disposal
at the end of the trial, detection methods for the transgenic organism,
protection from unauthorized persons, and so on.
The data requirements for a field release in Germany are not fundamentally
different from those in the USA, but the American approach seems to be
more pragmatic and straightforward. In the USA, the procedures needed to
obtain a field release permit are a well-established routine, while in
Germany only a few permits have been issued so far. [50]
4.3 Field Trials with Transgenic Plants
Field trials with transgenic plants world-wide are registered by the Green
Industry Biotechnology Platform (GIBiP), an industry association of
European companies which actively use biotechnology for the improvement
of plant varieties. The advantage of the GIBiP database is that it applies
the same definition for each entry, thus allowing an accurate comparison
of the field trials in the 32 countries where field trials have been
registered. One field trial is defined in the GIBiP database as "a single
basic genetic strategy introduced into a single crop and tested in a
single country by one company or institution in one year". Patricia Ahl
Goy and John H. Duesing have analysed the information in the database and
draw the following conclusions from the review of known activities
world-wide from 1986 to 1993. [20]
Up to the end of 1993, 1025 field trials with genetically manipulated
plants (GMPs) have been conducted world-wide. From 1986 to 1991 in North
America and the European Union/European Free Trade Association (EU/EFTA)
the number of field trials was approximately the same. However, since
1992, North America has become leading area and accounts for 517 trials,
slightly more than half of the world�wide total. As - according to the
definition of "field trial" mentioned above - the field trials in different
states of the U. S. are counted as one trial, but those in two different
EU/EFTA countries as two, the number of field trials in the USA is
underestimated compared to the EU/EFTA. Additionally, in North America
more trials with multiple locations take place, and therefore the lead
of North America is even larger. Ahl Goy and Duesing estimate that in
1993 single trials on over 1300 sites took place in North America, as
compared to less than 200 in the EU/EFTA. Activity in Central and South
America (6 % of all trials) has increased since 1991, principally serving
as a counter season location to evaluate material also being tested in
the Northern Hemisphere.
The reasons why the EU/EFTA has fallen behind North America are unclear.
According to Ahl Goy and Duesing [20] they could include
differences in agricultural crops (many crops important in America like
soybean, cotton, maize, flax and tomato are transformable since several
years, while important crops in Europe like wheat, barley and sugar beet
are more difficult to transform), the lower involvement of the European
public sector in research with transgenic plants (see below), and in
differences in the procedures for getting authorizations or
approvals.
38 different transgenic plant species have been field tested. The model
plant tobacco has been overtaken by crops of greater economic value. The
five crops potato, oilseed rape, tobacco, maize and tomato total more than
100 trials each and constitute together 70 % of all trials. The other
species tested up to the end of 1993 are sugar beet, soybean, cotton,
alfalfa, cantaloupe, flax, poplar, squash, rice, birch, chicory, lettuce,
petunia, cucumber, apple, cauliflower, sunflower, walnut rot, chrysanthemum,
gerbera, sugarcane, asparagus, brassica sp., cabbage, eggplant, eucalyptus,
kiwi, papaya, peanut, plum tree, spruce and strawberry. Given their economic
importance, monocots such as rice and cereals are clearly underrepresented
among trials to date because routine transformation of monocots lagged
behind dicots. Genetically modified rice and maize were field tested only
in 1990. The proportion of monocots is thought to rise during the next
years.
About one third of all field trials with the five most often test plants
evaluated pest and disease resistance. Due to the early success of coat
protein mediated protection and Bacillus thuringiensis toxins, virus
resistance (16 % of trials with the five most frequently used crops) and
insect resistance (13 %) are the most often tested traits in this sector.
Despite the economic importance of fungal and bacterial diseases, the
number of field trials with fungal and bacterial resistance is still low
with 3 % and 1 %, respectively.
The most commonly evaluated trait in plants still is herbicide tolerance
(34 % of all field trials), with a decreasing trend relative to other
traits. Besides the fact that genes conferring tolerance were among the
first to be identified and transferred, the main reasons for this
dominance are (1) a number of companies wish to expand the potential
market for their herbicides, (2) herbicide tolerance serves as a
selectable marker for the in vitro selection of other traits and for
recovering the transgenic segregants in the field, and (3) herbicide
tolerance serves as a marker gene for various studies, e.g. in risk
assessments. Other field-tested traits are quality improvement
(ca. 20 % of all trials), and marker gene (ca. 10%).
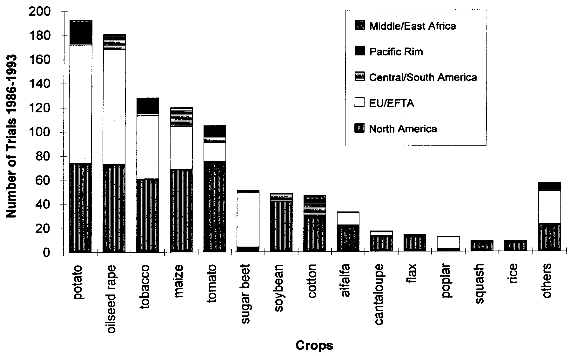
Fig. 4.1: Number of field trials with different crops world-wide
from 1986 to 1993. Calculated from data in [20]. Compare
also table 4.1
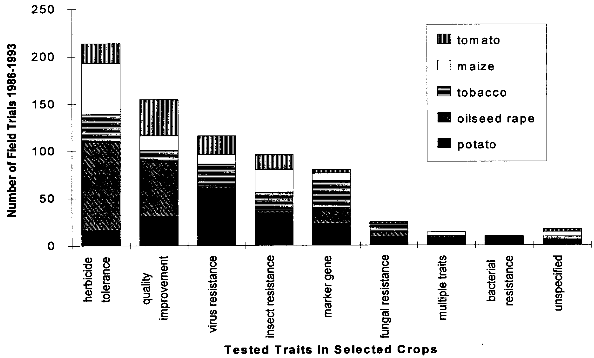
Fig. 4.2: Number of traits tested with the five most often genetically
altered crops. Calculated from data in [20]. Compare also
table 4.2.
The comparison of public and private activities shows that 61 public
institutions account for 29 % and 88 private companies for 71 % of all
known field trials. The public sector activity is higher in North America
(28 % of the notifiers) than in EU/EFTA (17 %). In the Pacific Rim, the
public sector is responsible for 79 % of the trials, while in Central
and Southern America 83 % of the trials are carried out by the private
sector (mainly counter season evaluation as mentioned above).
Table 4.1: Field trials with genetically modified plants according to the
crop and the geopolitical area
Genetically
manipulated
plant |
Number of field trials in |
North
America |
EU/
EFTA |
Central/South
America |
Pacific
Rim |
Middle/East
Africa |
Total |
potato |
74 |
| 98 |
3 |
16 |
2 |
193 |
oilseed rape |
73 |
95 |
12 |
0 |
1 |
181 |
tobacco |
61 |
52 |
2 |
13 |
0 |
128 |
maize |
69 |
35 |
15 |
0 |
1 |
120 |
tomato |
75 |
16 |
5 |
8 |
1 |
105 |
sugar beet |
4 |
45 |
2 |
0 |
0 |
51 |
soybean |
42 |
0 |
7 |
0 |
0 |
49 |
cotton |
29 |
0 |
11 |
3 |
4 |
47 |
alfalfa |
22 |
10 |
0 |
0 |
1 |
33 |
cantaloupe |
13 |
3 |
0 |
1 |
0 |
17 |
flax |
14 |
0 |
0 |
0 |
0 |
14 |
poplar |
2 |
10 |
0 |
0 |
0 |
12 |
squash |
9 |
0 |
0 |
0 |
0 |
9 |
rice |
7 |
0 |
0 |
2 |
0 |
9 |
others* |
23 |
27 |
2 |
5 |
0 |
57 |
Total |
517 |
391 |
59 |
48 |
10 |
1025 |
others* |
23 |
27 |
2 |
5 |
0 |
* others includes: birch (7), chicory (5), lettuce (5), petunia (5),
cucumber (4), apple (3), cauliflower (3), sunflower (3), walnut (3),
carrot (2), chrysanthemum (2), gerbera (2), sugarcane (2), asparagus (1),
brassica sp. (1), cabbage (1), eggplant (1), eucalyptus (1), kiwi (1),
papaya (1), peanut (1), plum tree (1), spruce (1) and strawberry (1).
Adapted from [20].
Table 4.2: Field trials with genetically modified selected crops according
to tested trait.
Trait |
Number of field trials with |
potato |
oilseed rape |
tobacco |
maize |
tomato |
herbicide tolerance |
16 |
94 |
29 |
54 |
21 |
quality improvement |
31 |
57 |
13 |
15 |
39 |
virus resistance |
60 |
2 |
24 |
10 |
20 |
insect resistance |
34 |
3 |
19 |
24 |
16 |
marker gene |
23 |
17 |
28 |
8 |
4 |
fungal resistance |
9 |
5 |
9 |
0 |
2 |
multible traits |
8 |
0 |
0 |
4 |
0 |
bacterial resistance |
9 |
0 |
1 |
0 |
0 |
unspecified |
3 |
1 |
5 |
5 |
3 |
Total |
193 |
181 |
128 |
120 |
105 |
Adapted from [20]. Disease and pest resistance in bold.
4.4 Commercialization
The release of transgenic crops is an important test for the efficacy of
the engineered traits under field conditions. If these tests are
successful and enough experience has been gained, an applicant may apply
for a commercialization permit. A permit is granted if the results of
field trials and laboratory tests have shown that production and
consumption of the transgenic crop does not pose risks for the consumers
or for the environment.
In the USA, the Animal and Plant Health Inspection Service (APHIS) must
confirm that the transgenic crop does not pose a plant pest risk and is
therefore not subject to regulation. In addition, the new variety must
be cleared by the Environmental Protection Agency (EPA) if the plant has
pesticidal traits, or by the Food and Drug Administration (FDA).
[47] In the European Union (EU), commercialization is
regulated under the directive 90/220/EC. If a permit for market
introduction is granted in one member state of the EU, the new variety
can be commercialized in all member states, but every member may comment
on the planned introduction. If one member state objects and no agreement
is reached, the European Commission or finally the Council of Ministers
decides on the commercialization. [50]
Several transgenic crop varieties have obtained a commercialization
permit. These include tomato, potato, maize, cotton, squash, soybeans,
flax, oilseed rape and tobacco varieties. The altered traits are extended
shelf-life, herbicide tolerance, insect resistance, virus resistance,
altered processing traits and modified oil profile (see table 4.4 for
details)
Table 4.4. Transgenic crops with commercialization permit granted.
Country |
Crop |
Trait |
Company or Institute/
Trade Name |
On the market/
Remarks |
USA |
Potato |
Insect resistance
(Bt toxin) |
Monsanto/
NewLeaf |
1995 |
USA |
Maize |
Insect resistance
(Bt toxin) |
Ciba Seeds |
1996 |
USA |
Squash |
Virus resistance
(viral coat protein) |
Asgrow/
Freedom II |
1995 |
USA |
Cotton |
Insect resistance
(Bt toxin) |
Mycogen Plant
Sciences/Bollgard |
Premarket
registration |
USA |
Tomato |
Extended shelf-life |
Calgene/
FlavrSavr |
1994 |
USA |
Tomato |
Extended shelf-life |
DNAP/
Endless Summer |
1996 |
USA |
Tomato |
More pectin/less water
(enhanced process value) |
Zeneca |
1996 |
USA |
Soybean |
Herbicide tolerance |
Monsanto/
Roundup Ready |
1995 |
USA |
Cotton |
Herbicide tolerance |
Calgene/
Laurical |
1995 |
USA |
Oilseed rape |
Altered oil composition |
Calgene/
Laurical |
1995 |
Canada |
Flax |
Herbicide tolerance |
University of
Saskatchewan |
1994 |
Canada |
Oilseed rape |
Herbicide tolerance |
AgrEvo/Monsanto |
1995 |
France/EU |
Tobacco |
Herbicide tolerance |
SEITA |
1994 |
Sources: For USA [51], for Canada [52] and Canadian
Biotech News on Internet, for EU [53]
As shown in table 4.4, the great majority of transgenic varieties have
been commercialized in the USA. In the European Union (EU), only one
variety has obtained a permit for commercial sales (herbicide tolerant
tobacco), but apparently this product has not been placed on the market.
An additional permit for herbicide tolerant swede rape in the EU is
expected.
All commercialized insect-resistant crops have been engineered with
Bacillus thuringiensis (Bt) δ-endotoxin genes. The potato variety
is resistant to Colorado potato beetle, cotton to the cotton bollworm,
tobacco budworm, and pink bollworm, and maize to the European corn borer
and other moth pests. The European corn borer alone is estimated to
cause some US$ 1 billion in crop losses annually to U.S. farmers. The
market introduction of these crops has been supported by EPA officials
because of the opportunity to reduce chemical-insecticide risks.
[54]
The transgenic virus resistant squash variety has been transformed with
viral coat proteins from the two squash pathogens watermelon mosaic virus
2 and zucchini yellow mosaic virus. The new variety now resists infection
by these two viruses. [55]
It is still to early to tell whether the transgenic varieties now present
on the market will be an economic success. The first genetically
engineered food on the market, Calgenes FlavrSavr tomatoes, trademarked
as MacGregor tomatoes and labeled as "grown from genetically engineered
seeds", have reached the first groceries during summer 1994. As tomatoes
soften and rot very quickly, they are usually picked when still green
and artificially ripened using ethylene gas before selling. The Calgene
tomatoes can ripe naturally because they soften more slowly and are then
transported to the stores. MacGregor's tomatoes were found to be slightly
better than supermarket tomatoes by the U.S. magazine Consumer Report,
but not enough to justify their premium price. [56]
It is very likely that the number of commercialized transgenic crops will
rise steadily during the next years. In the field of pest and disease
resistance, protection against various insect pests by expression of Bt
genes and coat protein-mediated protection against viruses in different
crop species will continue to be the most often engineered traits in the
beginning. Within some years, probably also varieties with enhanced
resistance against fungi (e.g. mediated by chitinases and other
pathogenesis related proteins from plants) and bacteria (e.g. conferred
by cecropins or antimicrobial proteins from plants) may become available.
Other applications of transgenic plants expected to reach the marketplace
within a few years include cotton producing altered fibers, crops with
improved nutritional value, and plants producing cheap vaccines,
pharmaceuticals or biodegradable plastic. [57]
|